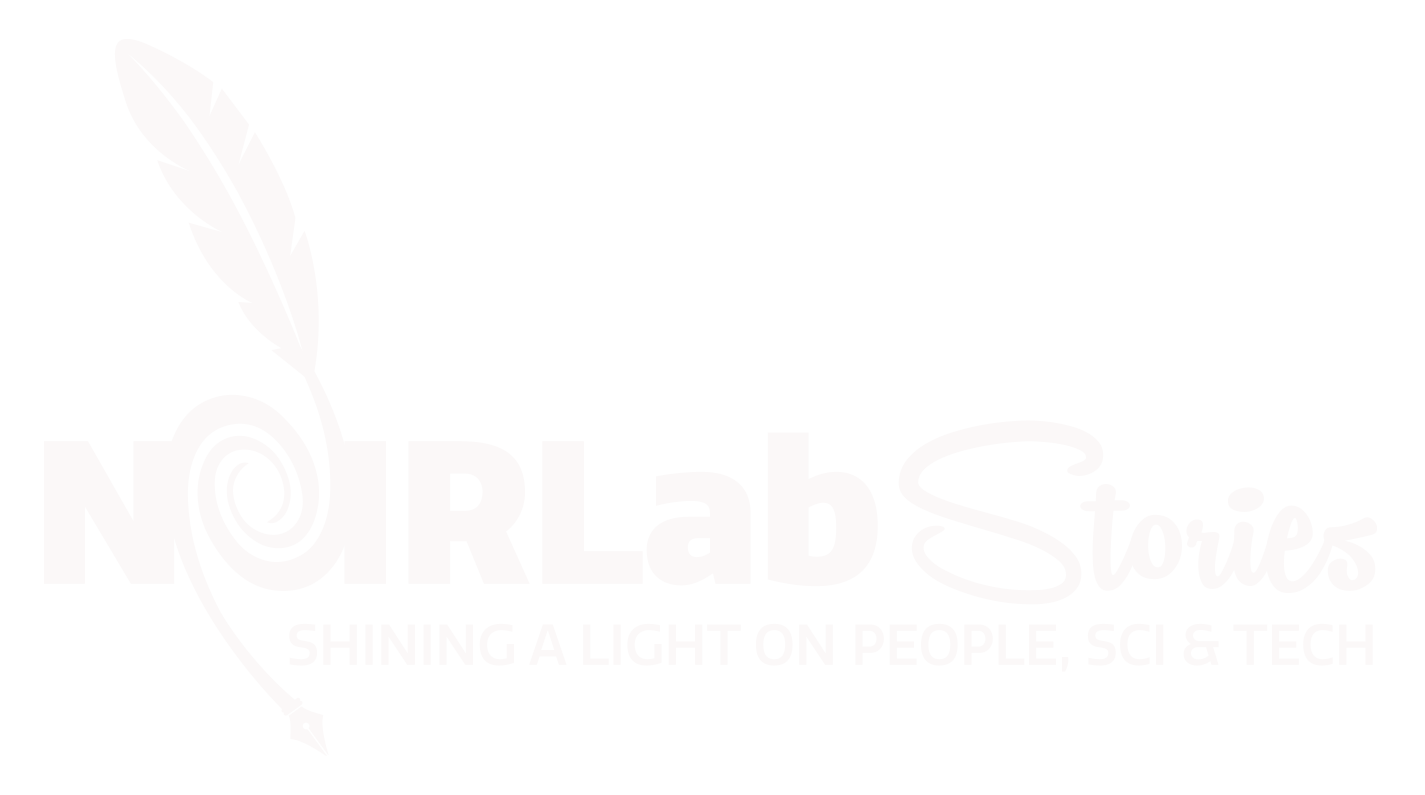
The UArizona 12-meter Telescope at Kitt Peak Is Part of Something Much Bigger: the Event Horizon Telescope
Radio telescope at Kitt Peak National Observatory helps explore the most extreme objects in the Universe
Profile
Name:
- UArizona 12-meter Telescope
Location:
- Kitt Peak, Arizona
Optical design:
- Cassegrain
Primary mirror diameter:
- 12 meters
Operational waveband:
- Radio
First light:
- 2014
Altitude:
- 1914 meters
Science goals:
- The telescope’s scientific program has evolved from one dominated by observing programs in astrochemistry to one with a broader mix of studies of molecular clouds and galactic star formation, evolved stars, astrochemistry, and external galaxies
8 June 2023
Mingled among the many domes and buildings at Kitt Peak National Observatory in Arizona are a couple of radio dish antennas. Apart from their obvious differences in design and appearance, these two antennas have a remarkable capability that other telescopes on Kitt Peak do not — they don’t just work alone. With the aid of atomic clocks and special processing, they can also become part of much larger telescopes thanks to an observing technique known as Very Long Baseline Interferometry (VLBI). One of the two telescopes, the Very Long Baseline Array Dish, is a part of the Very Long Baseline Array that stretches from Hawai‘i in the west to the Caribbean island of St. Croix in the east.
The other antenna is the University of Arizona 12-meter Telescope which, during normal operations, studies the sky on its own. When called upon, however, it can link up with other telescopes around the globe to become one of the newest additions to the aptly named Event Horizon Telescope (EHT).
Into the Edge of the Unknown
The EHT consortium made headlines in 2019 when it released the first-ever image of a black hole in the center of the massive elliptical galaxy Messier 87. The sensation of beholding Messier 87’s heart ultimately evokes a sense of wonder as it does an even deeper feeling of mystery: how do you exactly make an image of something as elusive as a black hole?
“The EHT has overcome those limitations, opening a new window on how we can study these ultradense cosmic enigmas through their event horizons.”
For the EHT, this seemingly impossible endeavor was achieved by harnessing an expansive network of observatories linked together to form a single virtual telescope. To better understand how this feat was accomplished, we first need to take a deep dive into the mind-boggling physics of a black hole.
The Shadowy Hearts of Galaxies
Black holes come in several varieties. The vast majority are stellar-mass black holes, which result from the collapse of a star at least twenty times the mass of our Sun. As the star goes out with a ‘bang’ during a supernova, the density of its crumbling core creates a gravitational well so great that nothing can escape, not even light. The boundary of no return that forms afterwards is called the event horizon.
At the other end of the scale are supermassive black holes, which can be many billions of times more massive than our Sun. They reside in the centers of galaxies, such as the black hole at the center of the Milky Way known as Sagittarius A* (or Sgr A*, with the asterisk pronounced as ‘star’). The extent of an event horizon is proportional to the black hole's mass, so while a stellar-mass black hole’s would be minuscule in comparative size, the event horizon of one like Sgr A* would be on the order of about 12–24 million kilometers across. An even larger supermassive black hole, like the one in Messier 87, can span up to a remarkable 3000 times larger than the black hole at the center of the Milky Way.
The extraordinary influence of supermassive black holes on their host galaxies is what makes them such prime subjects for study. Prior to the EHT observations, black holes could only be observed indirectly, either by the motion of nearby objects — like stars orbiting the Milky Way’s center — or by the energy released when they feed on infalling matter. The EHT has overcome those limitations, opening a new window on how we can study these ultradense cosmic enigmas through their event horizons. By using a special technique called Very Long Baseline Interferometry (VLBI), it is possible to resolve supermassive black holes even from a great distance here on Earth.
VLBI and the Event Horizon Telescope
VLBI works by combining signals received from a collection of radio dishes separated by as little as a few tens of meters or as much as thousands of kilometers. This network of telescopes can then function as a single virtual telescope the size of the maximum distance between the dishes. The greater the distance between the antennas, the larger the virtual telescope and the greater its resolution.
This technique was utilized by the Event Horizon Telescope collaboration, which was founded in 2009. Its purpose is to harness an interconnected network of telescopes with the resolution necessary to image the event horizon of a supermassive black hole. What makes the EHT different from other radio telescope arrays is its enormous geographical scale and its ability to observe at relatively short wavelengths.
The shorter the wavelength, the higher the resolution. Shorter wavelengths, however, pose technical challenges and require clear observing conditions at every element of the telescope at the same time. Poor conditions at just one location could thwart an observation. Yet when conditions are just right, the EHT, observing at radio wavelengths of 1.3 millimeters, has the resolving power equivalent to imaging the date on a quarter in Los Angeles all the way from New York! This was the level of precision needed to capture the black hole in the center of Messier 87.
Though even with its robust set of capabilities, the EHT suffers from the same issues inherent in all interferometers: spatial coverage and sensitivity. When astronomers say the EHT is a virtual Earth-size telescope, that’s not quite the whole story. The array gets its resolving power from the extreme separation of its individual parts; but with this separation comes gaps in the data. Furthermore, the sensitivity of the telescope is a result of its total collecting area, and despite its tremendous geographical reach, the individual EHT dishes do not take up that much real estate.
“VLBI is a funny thing,” says University of Arizona radio astronomer Dan Marrone. “You take data and you record it, but you have no way to know whether the data is any good until months later.”
Another challenge is bringing all the data together. The distances involved and the vast amounts of information collected make normal data transfer via the internet impractical. The data would have to be recorded at the various sites, and then shipped to a central location where powerful computers can merge them together.
“VLBI is a funny thing,” says University of Arizona radio astronomer Dan Marrone, who is a member of the EHT science council. “You take data and you record it, but you have no way to know whether the data is any good until months later when you multiply it against some other telescope and see if you saw the same [radio waves] arriving… there are a million ways you could screw that up.”
For interferometers, like the Very Large Array (VLA) in New Mexico and the Atacama Large Millimeter/submillimeter Array (ALMA) in Chile, it is possible to overcome that issue to some extent by observing for longer periods of time: hours, to even days, in total. As Earth rotates, parts of the array rotate as well and slowly fill in these gaps, like a Spirograph™ rendering more and more of an image the longer your pen spins in its gears. The EHT, however, does not have that luxury of time.
To alleviate this issue, the EHT is constantly looking to expand its network. One important addition is the UArizona 12-meter Telescope at Kitt Peak National Observatory.
The UArizona 12-meter Telescope
In 2013, the University of Arizona received a 12-meter millimeter-wavelength telescope, which was originally created as one of the prototype antennas for ALMA that underwent testing at the VLA site. After the prototypes passed muster, they were made available for independent research as standalone telescopes. This one found its way to Kitt Peak National Observatory in Arizona as an independently operated tenant telescope.
To properly function at Kitt Peak, however, changes needed to be made. A stronger foundation was built for more precise pointing, a new receiver system was designed to better suit the antenna’s capabilities, and new equipment was installed for VLBI purposes, including an atomic clock. Despite taking an additional several years to complete, the renovation of the ALMA antenna would prove to be integral in the telescope’s partnership with EHT.
“Having the UArizona 12-meter Telescope essentially mends together any of the puzzle pieces that EHT had been missing.”
The UArizona 12-meter Telescope started its EHT testing runs in early 2021, after being delayed by the pandemic in 2020. In order to properly test the telescope, Marrone and his team pointed it at one of the brightest radio objects in the sky, a quasar named 3C 84. The tests were successful, and by 9 April 2021, the UArizona 12-meter Telescope was officially part of the EHT. Performing well during the EHT observing season in 2022, it will continue to collect data for years to come.
Scoping the Future with EHT
Even with added elements, observations with the EHT and similarly configured interferometers still suffer from a dearth of coverage. In essence, the total is not necessarily the sum of its parts, and the gaps in coverage mean the images may not be as sharp as astronomers would like. A newly developed machine-learning technique was recently applied to the original data from the EHT observations of Messier 87. The result is a clearer image that can help astronomers better understand the properties of an event horizon. To learn more, read this recent news release.
There is still a copious amount of information we don’t know about black holes, but with the inclusion of a new radio dish at Kitt Peak National Observatory, our perspective of the Universe has been sharpened — literally. Every new addition to EHT counts.
The benefit of Kitt Peak’s collaboration is its relatively close proximity to some of the EHT’s other telescopes: the Submillimeter Telescope on Mount Graham, Arizona; and the Large Millimeter Telescope in Sierra Negra, Mexico. Having the UArizona 12-meter Telescope essentially mends together any of the puzzle pieces that EHT had been missing, including the jet emissions of a black hole that are too large in size for its earlier array to capture. Moreover, the improved clarity of the EHT encourages scientists to not only probe for new black holes, but revisit previously imaged ones as well.
Having studied Sgr A* for over 20 years, Marrone explains that the supermassive black hole at the center of the Milky Way has a perceived ‘flicker’ that confounds many radio astronomers. This change in brightness occurs so rapidly that some speculate it must be happening close to the event horizon. Increasing the EHT’s roster will help catch more accurate observations of any apparent movement around the black hole, as the imaging capability depends on the number of pairs of telescopes operating at once. Each pair introduces new information about the object with much more clarity for every telescope addition.
“We need at least five observatories at different places observing at the same time in order to get an image,” Marrone remarks. “Adding Kitt Peak to the array means that we get additional observing time each night, which means that we can see Sgr A* changing as things circle the black hole.”
Scientists have yet to fully understand the mechanism behind a black hole’s jet emissions, how they change over time, and many other fascinating characteristics; but by capturing the entire picture, we may gain unexpected insight that is much more profound than we could possibly imagine. Having the whole context is indispensable when facing a perplexity like a black hole, and with the UArizona 12-meter Telescope at Kitt Peak National Observatory as part of the endeavor, it certainly brings the astronomy community one step closer.