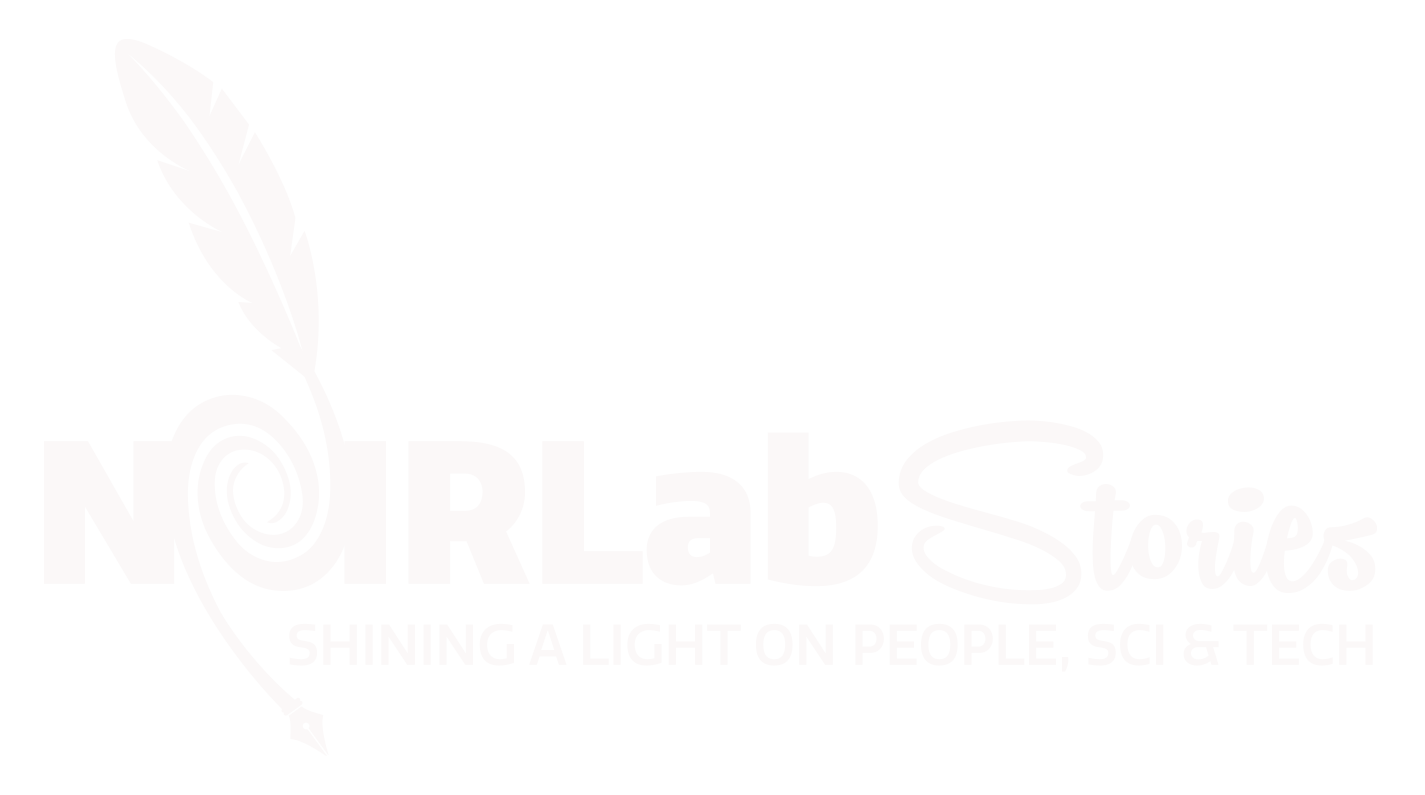
How quickly is the Universe expanding?
A NOIRLab astronomer has helped provide more evidence of a puzzling discrepancy in the measurement of the expansion rate of our Universe
Profile
Name:
-
Hubble Space Telescope
Operator:
-
NASA, ESA, Space Telescope Science Institute
Optical capabilities:
-
Ultraviolet through to infrared
Launch date:
-
April 1990
Primary science goal:
-
Peering into the observable Universe so that scientists can better understand it
24 Aug. 2021
Across cosmic history, the Universe’s expansion rate has been increasing, thanks to the accelerating effect of dark energy, and so the Hubble “constant” (H0) is only actually constant for any one period in time. However, there is a growing tension between what the value of H0 in the Universe today is predicted to be by the science of the early Universe, and local measurements of H0 calculated with the use of distance markers such as Cepheid variable stars, Type Ia supernovae and red giant stars. It's a bit like looking at a picture of yourself as a baby, and then at a picture of yourself as an adult, and then working out how fast you grew to be your current size.
“If all the different routes converge on a value of H0 in the late universe that disagrees with the predicted value based on the early Universe, then the tension is real and is telling us that the Universe is more wonderful than we had imagined,” says Blakeslee.
No matter where you are in the Universe today, you would see the cosmos expanding at the same speed.
Astronomers have been attempting to measure H0 more precisely ever since 1929, when Edwin Hubble discovered that the Universe was expanding. Independently he and the Belgian mathematician and physicist Georges Lemaître developed a simple mathematical equation to describe this expansion, known as the Hubble–Lemaître Law. It goes as follows: the velocity at which a galaxy is being carried away from us by cosmic expansion is equal to H0 multiplied by the distance to that galaxy. You may have seen it written in shorthand as v = H0 d.
H0 is written in units of kilometers per second per megaparsec (km/s/Mpc; one parsec is 3.26 light-years, and a megaparsec is a million parsecs). Blakeslee’s team have concluded that H0 is 73.3 km/s/Mpc, which if correct means that every megaparsec of space is expanding by 73.3 kilometres every second.
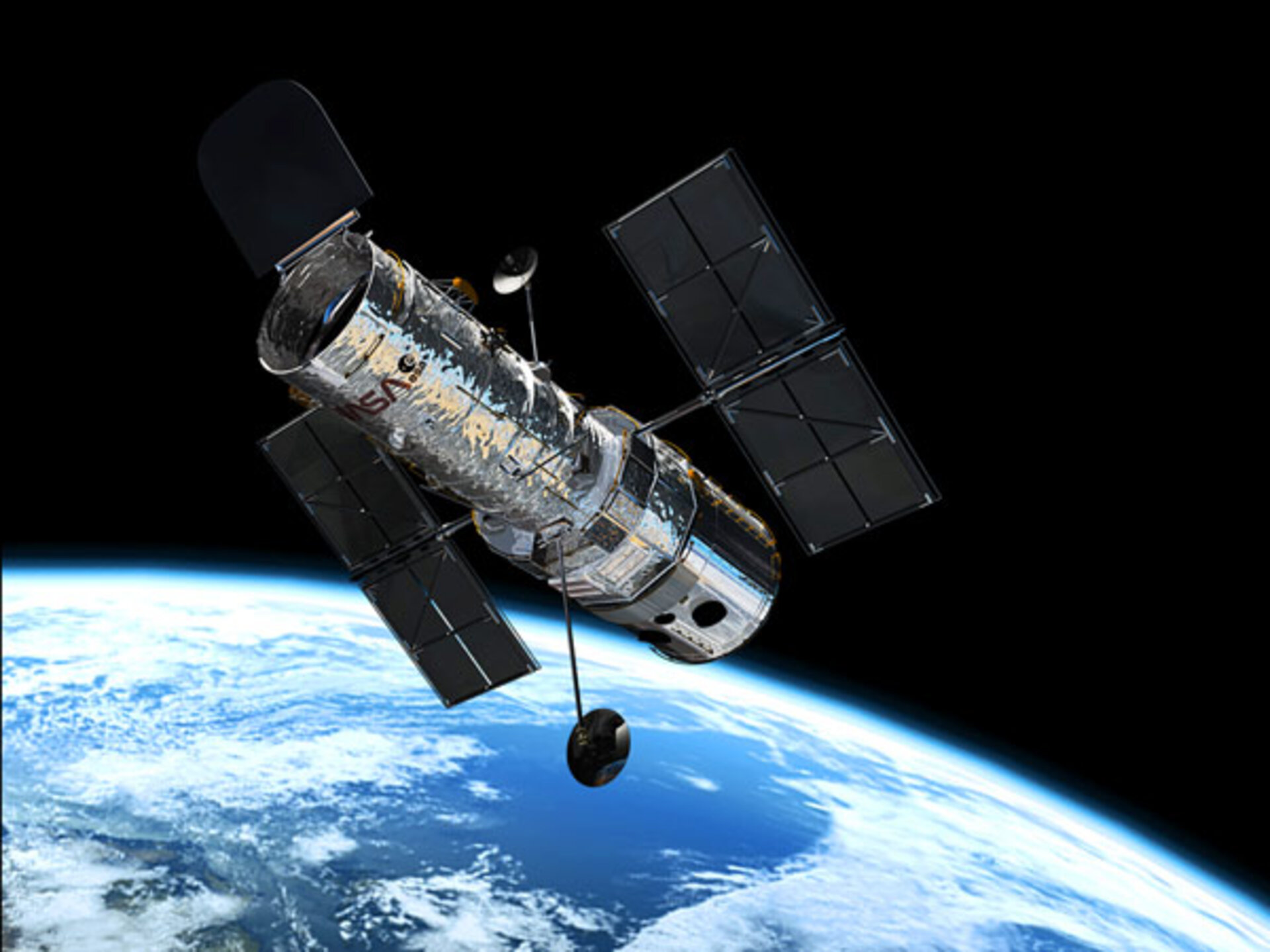
It’s been a long journey for astronomers to be able to make accurate measurements of H0. Early attempts, going back to Edwin Hubble’s own measurements in 1929, were inaccurate partly because of limitations in telescopic capabilities, but also because of an incomplete understanding of standard 'candles'.
Astronomers use standardizable 'candles' to measure distance across large expanses of space. They include the aforementioned Cepheid variables, Type Ia supernovae and red giant stars. They are referred to as standardizable because their peak luminosity is relatively easily predicted, and if we know how intrinsically luminous a celestial object is, astronomers can judge how far away it must be based on how bright it appears to us. Meanwhile, the velocity at which a galaxy is moving away from us with the expansion of space can be worked out from its redshift.
Edwin Hubble’s own estimate of H0, in 1929, was 500 km/s/Mpc, but confusion between different types of Cepheid variable stars skewed his value. Yet his measurement stood for over 20 years, until observations by the 200-inch Hale Telescope on Palomar Mountain brought things into focus. Astronomers began to settle into one of two camps: those, led by Allan Sandage, who argued for a value of H0 nearer 50 km/s/Mpc, and those such as Gerard de Vaucouleurs who believed it to be higher, at 100 km/s/Mpc.
Astronomers have been attempting to measure H0 more precisely ever since 1929, when Edwin Hubble discovered that the Universe was expanding.
Over time, the measurements began to meet in the middle, and by the turn of the century expectations were that H0 was somewhere between 65 and 80 km/s/Mpc, but the measurements were still too imprecise to be able to pin it down any further.
Then came the European Space Agency’s Planck mission, which observed the cosmic microwave background (CMB) radiation between 2009 and 2013, providing astronomers with the most detailed observations of the CMB yet made. By applying our best models of cosmology to understanding the features seen in the CMB, scientists were able to extrapolate from that and predict what the value of H0 should be today. Planck found, with an uncertainty of less than 1%, that H0 is 67.4 km/s/Mpc.
Hot on the heels of Planck’s measurement came a huge twist. In 2016, using Cepheid variables to calibrate distance measurements of Type Ia supernovae, astronomers led by Adam Riess of Johns Hopkins University calculated H0 to be 73.2 km/s/Mpc, with an uncertainty of less than 2%.
There’s no overlap between the uncertainties in the two measurements, and no obvious indication of where a mistake may have been made; so why are the values of H0 different?
The findings have been further compounded by the fact that other measurements of the early Universe side with Planck’s findings, while more local measurements of stars and galaxies side with the supernova measurements. The implication is that there could be something amiss in our understanding of the Universe.
The implication is that there could be something amiss in our understanding of the Universe.
Blakeslee’s group has provided further evidence for this split in values. To obtain their value of H0 of 73.3 km/s/Mpc, they looked through archive data from the Hubble Space Telescope’s Wide Field Camera 3 (WFC3) to study 63 giant elliptical galaxies out to a distance of 100 Mpc, looking for surface brightness fluctuations (SBF) in the galaxies’ overall brightness, and then using the SBF to judge the distance to each galaxy.
“Because the red giant stars that primarily cause the fluctuations in elliptical galaxies emit most of their light in the near-infrared, the method becomes much more powerful using the high-quality, space-based infrared data from Hubble's WFC3,” says Blakeslee. “It took us several years to build up a sufficient sample and analyze all the data, but now we have by far the best-ever measurement of H0 from SBF.”
Their value of H0 is very close to the value obtained from Type Ia supernovae measurements and studies of gravitationally-lensed quasars, but this doesn’t mean it is case closed. Many more measurements are required to improve the chances that these measurements are correct, and not some error or statistical fluke. Given that there are many more elliptical galaxies than Type Ia supernovae, the SBF method can play a large part in that by vastly expanding the number of data points.
Currently, the precision of the SBF method in measuring H0 is a little over 3%, compared to the 2% for Type Ia supernovae and 1% for Planck. However, observations by the forthcoming James Webb Space Telescope, when combined with astrometric measurements from the Gaia spacecraft, should reduce the precision of SBF to better than 2%.
“At that point," says Blakeslee, "The Hubble constant tension either will have vanished or, as is looking increasingly likely, will be proof of new fundamental physics.”